Deep ocean outfalls: A sustainable solution for Sydney
By P Tate and C Marvell.
First published in Water e-Journal Vol 1 No 2 2016.
Introduction
There is no universal solution to “the best” option for the disposal of wastewater. Any solution adopted should incorporate an appropriate level of technology combined with consideration of the environment into which the wastewater will be discharged. Further, it is necessary to consider the overall “energy penalty” associated with any solution. Inevitably, this means unique solutions for individual outfalls.
Roberts (2010) asserts that ocean “outfalls are often perceived poorly in the eyes of the public and political officials”. He points out that properly designed wastewater treatment and disposal systems provide an effective strategy while also minimising environmental impacts.
For Sydney’s three deepwater ocean outfalls (DOOs), it is perhaps easy to now question decisions made in the 1960s and 1970s. Such decisions must be placed in the context of knowledge and technology available at that time. The lead-time between making a decision and commissioning a complex engineering structure may be decades. Nevertheless, it is important to continually assess whether those decisions are appropriate now and into the future. If they are not appropriate, then planning for alternative solutions should commence.
It is 25 years since commissioning of the first of Sydney’s deepwater ocean outfalls, the Malabar outfall, in September 1990. In this paper the authors revisit the process leading to construction of the deepwater ocean outfalls, assess the performance of the outfalls against their design criteria, and determine whether they are a sustainable option for the ongoing disposal of wastewater from the bulk of Sydney’s population.
The deepwater ocean outfalls
How we arrived at the DOO option
In the late 1700s, Sydney’s wastewater was discharged, untreated, into the local waterway – the Tank Stream. By the early 1800s it became apparent that pollution levels were unacceptably high (even by the standards of those times) and the discharge of wastewater was directed to Sydney Harbour. Again, it became apparent that the harbour was becoming increasingly polluted and a decision was made to discharge wastewater to the ocean via cliff-face outfalls at Bondi (commissioned in 1889), then Malabar (1916) and North Head (1926) (Hector, 2011). In the late 1800s daylight bathing at ocean beaches was banned and beach usage was considerably less than it is today.
As the population of Sydney grew, so too did the volume of wastewater being delivered to the coastal treatment plants and subsequent disposal to the ocean via cliff-face outfalls. Not only did this result in discolouration of the ocean waters, but high concentrations of indicator bacteria in the beach bathing waters became more frequent, as did the presence of “grease balls” on the beaches (Figure 1). The ability of the wastewater plumes to break up via natural processes was substantially reduced because of low dilutions the cliff-face outfalls achieved.
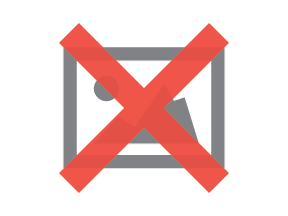
From the 1960s onwards, a number of wastewater treatment and disposal options were considered as part of a long-term solution to environmental pollution. By the 1970s, four basic options emerged:
- Increase wastewater treatment and reuse. There were no local industries to use the volume of wastewater produced, hence there was a need to transport the wastewater to those locations where reuse could take place. This relocation involved large cost, traffic congestion and likely odour problems.
- Secondary treatment (activated sludge) and shoreline discharge. There was insufficient land available for the secondary sedimentation tanks. Costs to acquire the necessary land were prohibitive.
- Advanced primary treatment and nearshore outfalls for disposal. Indications were that plumes would still be visible for much of the year. Further, adding chemicals to treat the wastewater could result in (unforeseen) environmental problems.
- Deepwater ocean outfalls and primary treatment. This was deemed the best overall option and was selected in 1980 as the way forward.
It was also recognised that: “Because of the established nature of the Sydney sewerage systems, it would not be feasible to consider significant changes to the basic system layout” (CCE, 1976). The sewerage system then (and now) uses gravity as the fundamental means for moving wastewater and is highly efficient in the energy it expends. To change this system and redirect flow inland would require extremely large pumps, pressurised pipelines, exorbitant pumping costs and a large environmental penalty through the use of fossil fuels. In the 1970s, when these decisions were made, alternate (to fossil fuels) energy systems were either not available or inefficient.
During the 1980s, there was a groundswell of discontent opposing the ocean disposal of wastewater among Sydney’s population in general and the coastal communities in particular. The “POOO marches” (People Opposed to Ocean Outfalls) were commonplace in the years prior to the commissioning of the deepwater ocean outfalls. Evidence of sewage pollution on Sydney’s ocean beaches was clear – typically characterised by a brown stain of the water surface and grease balls on the beaches that sometimes reached the size of cricket balls. This often occurred during summer (and correspondingly high beach usage), when the prevailing onshore winds were responsible for moving the wastewater plumes towards the ocean beaches.
Design criteria
The Environmental Impact Statement (EIS) made predictions on the likely performance of the outfalls, based on preliminary studies between 1972 and 1975 (CCE, 1976). CCE analysed data collected from a range of studies, including:
- Determining current patterns and the density structure of the ocean waters.
- Characterising the chemical composition of the seawater and sediments.
- Establishing baseline conditions against which the performance of the outfalls could be compared. Studies included soft and rocky bottom communities, fish communities, seabirds and faecal coliforms in the beach bathing waters.
- Special studies included geological surveys and dispersion and mixing studies.
The EIS predictions formed the basis of the design criteria. The design flows were based on 2025 projections. The design criteria are summarised below:
- Dilutions lower than 40:1 should occur less than 2% of the time;
- Wastewater plumes to be submerged >90% of the time in summer (1 November to 1 May each year);
- Faecal coliforms in beach bathing waters < 200 cfu/100mL – calculated as a monthly geometric mean;
- During summer (1 November to 1 May each year), 90% of samples from beach bathing waters should have faecal coliform concentrations <400 cfu/100mL;
- During winter (1 May to 1 November each year), between 9am and 9pm, concentrations of faecal coliforms in beach bathing waters <2,000 cfu/100mL.
Originally, the outfalls were designed to include sludge disposal. However, this option has never been exercised and is unlikely ever to be used, as the biosolids now are 100% reused by agriculture.
Based on the information it collected and analysed, CCE (1976) concluded that “the submarine outfall design concepts recommended in this report will not cause any significant adverse effects on the marine environment resulting from the disposal of treated wastewater”.
All three outfalls have the same basic configuration, the main differences resulting from different populations, hence different flows. Each outfall has the potential for future growth. The opportunity for expansion is limited at Bondi, moderate at Malabar, but substantial at North Head.
Three basic options for the new outfalls were short-listed:
- Vertical shaft and short tunnel, trenched through the surf zone (concrete or steel pipeline);
- Tunnel to about half distance, then complete the outfall with a trenched pipeline;
- Tunnel the entire distance of the outfall, then use multiport risers from the last ‘x’ metres to form the diffuser section.
The last of these options was selected, mainly on the basis of ease of construction and the energy needed to move the wastewater through the tunnel.
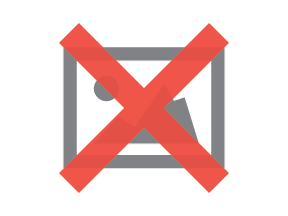
While the detail of each outfall is unique (Table 1), the broad configuration for all outfalls is the same, comprising a drop-shaft from the treatment plant to about 130m below the sea surface (Figure 2).
The outfall tunnel (housing the wastewater pipeline) extends from the bottom of the drop-shaft, sloping upwards to ensure that any air entrained does not remain in the pipeline. The final several hundred metres of the outfall pipeline comprise the diffuser section. Tapering of the pipeline ensures that wastewater velocities are maintained, reducing the likelihood of particulate matter settling in the bottom of the pipeline.
In the diffuser section, long risers (about 50m) extend from the pipeline to about 2m above the sea floor. Each riser terminates in a multiport diffuser (Figure 3) that discharges wastewater horizontally through outlet ports about 100mm in diameter (the diameter differs for different outfalls and risers). Wastewater flow to the outfalls is by gravity, although some pumping is required to deliver wastewater to the treatment plants.
The design of the deepwater outfalls is relatively simple. They are self-cleansing and have no moving parts, substantially reducing maintenance costs. Ongoing maintenance comprises six-monthly inspections of each outlet port by ROV, ensuring wastewater flows through all outlets as designed. Outlet port blockages are rare, although a safety hat and a small portion of tunnel lining were found to inhibit flow through two separate outlets on two separate occasions. There are occasional build- ups of solids that block the outlet ports, but these are released by a purpose-built robotic tool after identification during routine monitoring.
- Previous overseas outfalls had encountered problems that rendered them inefficient. The design of the DOOs solved many of these problems, including: entrainment of air into the pipeline, seawater intrusion, sedimentation and marine growth, all of which have the potential to reduce the efficiency of the outfall:
The upwards slope of the pipeline helps prevent air that may have been entrained into the pipeline via the drop shaft from remaining in the pipeline. Eventually, any entrained air will move through the pipeline and exit via the outlet ports.
- Under certain environmental conditions seawater may enter the pipeline, potentially reducing the operating efficiency of the outfall. As part of the operating design, a procedure was developed to purge seawater from the pipeline. Generally, the problem occurs during high spring tide water levels and the head available to drive the wastewater flow is low. The procedure can be implemented at low tide levels or when the spring tide phase has passed.
- Wastewater composition includes particulate matter. Under low wastewater flow, there is a potential for this particulate matter to settle in the bottom of the pipeline. To maintain the velocity of wastewater and help prevent sedimentation, the diameter of the pipeline in the diffuser zone is reduced as the distance offshore increases.
- Marine organisms can attach to the outlet ports and excessive growth may impede wastewater flow. High velocity discharge of wastewater inhibits such growth. In addition, sea anemones have taken up residence on the diffuser heads and they help keep the heads free of any crustaceans.
How the outfalls work
Dilution and entrainment of the wastewater plume
Wastewater from the DOOs is discharged as a buoyant jet – that is, at velocities much higher than the surrounding ocean currents and at densities much lower than that of the ocean waters. These two factors cause ocean waters to be entrained into the wastewater plume. The velocity difference between the wastewater and the ocean waters causes shear between these two fluids (Figure 3). The shear causes instabilities at the interface between the two fluids. As these instabilities grow, they engulf (or entrain) ocean water into the wastewater plume, which then becomes more dilute.
The lower density of the wastewater (compared with the surrounding ocean water) causes the wastewater plume to rise through the water column. As the plume rises, it pushes through the ocean water above. This vertical movement of the plume causes a small wake on the underside of the plume, resulting in vortex-type flow. Ocean water enters the plume (or is entrained) by this vortex flow and the wastewater plume is diluted (Figure 3).
These two entrainment processes cause the wastewater plume to eventually have velocity and density properties close to that of the ocean waters. When that occurs, the vertical movement of the plume ceases. For Sydney’s DOOs, this process takes about 10 minutes and occurs within about one-to-two hundred metres of the discharge point (although these values can vary considerably, depending on oceanic conditions). Further dispersion of the wastewater occurs via oceanic turbulence. In general, 90% of the wastewater dilution occurs via the entrainment process.
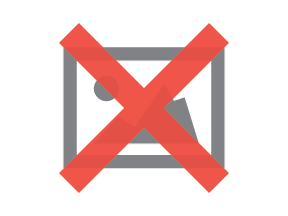
Effects of ocean currents
Ocean currents enhance dilution, enabling the organic material in the wastewater to be more easily broken down. Further, it has been known for many years that saltwater acts as a mild disinfectant, inactivating many viruses (e.g. Lycke et al., 1965). The dominant current system off Sydney is the pole-ward flowing East Australian Current (EAC). This is an example of a western boundary current (WBC) and similar systems occur along the western boundaries of all oceans (e.g. the Gulf Stream in the North Atlantic Ocean). WBCs are the intensification of ocean waters along the western boundaries of all oceans due to the rotation of the Earth.
The mainstream of the EAC lies about 100km offshore from Sydney and it is only the western edge of this current into which wastewater from the DOOs is discharged. The current system is complex and highly variable. While currents in the mainstream EAC often reach speeds exceeding 2 m/s, the currents near the DOOs are typically in the range 0.2-0.5 m/s, although they can reach speeds of 1 m/s or more. The movement of the EAC is regularly mapped by CSIRO and an example is shown in Figure 4. Generally, the EAC moves southwards along the NSW coast, breaking away from the coast north of Sydney then meandering into the Tasman Sea, where it eventually dissipates.
Stratification
Stratification of the water column governs the height to which the wastewater plumes rise in the water column. If the plumes reach the surface of the ocean they may become visible and, under appropriate wind conditions, they may move towards the ocean bathing beaches. Stratification is simply the variation in density throughout the water column, driven by variations in temperature and salinity. In summer, solar heating of the surface waters lowers its density compared with deeper waters, and the waters become thermally stratified. Generally, a temperature difference of 1oC over 50m is sufficient to produce a submerged wastewater plume. This occurs more than 95% of the time. It is only during the coldest time of the year or when large storms mix the whole water column that the stratification breaks down and the wastewater reaches the surface of the ocean.
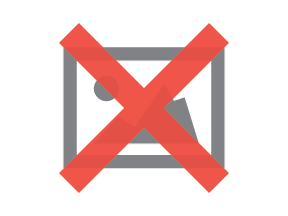
Since 1990, Sydney Water has managed an ocean monitoring system designated the Ocean Reference Station (ORS), which captures current speed and direction data as well as temperature (and some salinity) data throughout the water column. The data collected are used as input to numerical models that estimate the movement and dilution of the wastewater plumes discharged through the three deepwater ocean outfalls. The models (Tate and Middleton, 2000, 2004) have been calibrated and validated using both laboratory studies (Couriel and Wilkinson, 1993) and a set of extensive field experiments in which radioisotopes were injected into the wastewater and its movement and dilution tracked after exit from the deepwater ocean outfalls.
The ORS underwent major reconfiguration in May 2006, and model results are compared with design criteria from May 2006 to June 2014. Results are compared with the design criteria and presented in Table 2 (for plume dilution) and Table 3 (for surfacing plumes). In all years, the model results indicate the outfalls perform better than designed. Perhaps this result is expected, as engineering designs are inherently conservative. Further, these results show no significant change through time, implying that the DOOs continue to operate as designed.
Environmental monitoring
The Environmental Monitoring Program
In 1986, a pilot study commenced aiming to identify components (and techniques) of work that would comprise an Environmental Monitoring Program (EMP). The main aim of the EMP was to quantify initial environmental impacts associated with the operation of the new deepwater ocean outfalls. The EMP was managed by the New South Wales Environment Protection Authority. It ran between 1988 and 1993 and resulted in many interpretative reports, mostly summarised in a special issue of Marine Pollution Bulletin (1996). A brief overview of the EMP results is provided in the following section.
The EMP was designed to address the following three questions:
- Will it be safe to swim in the beach bathing waters?
- Will it be safe to eat the fish and shellfish?
- Will the marine environment be protected for future users?
The basic philosophy in executing the EMP was to: (a) identify the types and concentrations of contaminants in the wastewater; (b) determine where the outfall plumes move and how dilute they become; and (c) quantify impacts on water quality and marine communities.
Routine monitoring of the wastewater was undertaken to identify the types, concentrations and variability of contaminants contained therein. The link could then be made between what is being discharged and the potential impact that these contaminants may have on the marine communities.
Oceanographic experiments (including advection/dispersion and plume dilution studies) were undertaken to understand the physical processes important to the movement and dilution into the development, calibration and validation of a suite of numerical models. Central to this understanding, and input to the numerical models, was data from the ORS.
Impacts of the DOOs were quantified by examining change in a broad range of systems and biological communities. The studies included:
- Ocean and beach bathing water quality;
- Contaminants in the sediments, biota and oceanic microlayer;
- Biological community studies – hard and soft seabed biota, plankton and demersal fish.
Summary of findings from the EMP
Results from the EMP indicated the early performance of the DOOs broadly met its objectives. Koop and Hutchings (1996) summarised the findings of the EMP, which included:
- “… the deepwater outfalls are performing well, i.e. that sewage plume dilutions exceed design specifications, and that they have reduced the degree of beach pollution in the Sydney area … (and) created no new problems in ocean waters near the deepwater outfalls”;
- “… demonstrating little detrimental environmental effect of the new mode of sewage disposal …”;
- “Although significant effects on some benthic taxa have been recorded … some taxa exhibiting changes, both increases or decreases … there have been no consistent patterns in the observed changes”;
- Also, they note that the previously degraded areas of the inshore waters recovered after the cessation of the shoreline discharges;
- Finally, they state that: “short-term effects of deepwater sewage disposal off Sydney are small and environmental impacts difficult to detect”.
However, Koop and Hutchings (1996) do argue that monitoring needs to continue to ensure that adverse impacts don’t manifest themselves years or decades into the future.
Philip and Pritchard (1996) state that the “outfalls have performed as well or better than was predicted at the time the EISs were prepared”. However, they do go on to note that the duration of the EMP was limited (compared with the anticipated life of the DOOs) and the long-term accumulation of contaminants in sediments and their effect on biological communities need to be monitored.
Ongoing monitoring
The EMP was followed by an ongoing monitoring program, which currently includes:
- Monitoring of the wastewater flows and hydraulic performance of the outfalls;
- Continued characterisation of the wastewater;
- A “whole-of-effluent” toxicity testing program;
- Continued operation of the ORS and running of the near- field plume models;
- Monitoring to assess the accumulation of contaminants in the sediments and whether changes in the structure of infaunal communities can be identified;
- Monitoring (by “Beachwatch”) of beaches and beach bathing waters for visual and bacterial contamination.
Hydraulic monitoring indicates no change from the design specifications in the operation of the outfalls. This is supported by the year-by-year assessment from the model output: the outfalls continue to operate better than the design criteria and there is no meaningful, temporal change in these characteristics.
Based on monitoring over the last 25 years, the following observations are made:
- The outfalls are operating as designed (or better);
- There are no toxic effects on test species in the wastewater at concentrations outside the licence limits;
- There has been no major shift in the dilution or movement of wastewater after discharge;
- No significant change in biota or concentration of contaminants has been observed in the sediments;
- There are no negative impacts in ocean beach bathing waters (enterococci) as a result of the DOOs.
Evidence from the environmental monitoring indicates that no statistically significant impacts have been found since the DOOs were commissioned. In the absence of clearly identified impact, we conclude that the DOOs are operating as designed and have no (or no meaningful) effect on the marine waters and communities off Sydney. Based on these findings, the outfalls are likely to be a sustainable option for the disposal of Sydney’s wastewater into the future. However, if there was a change in any of the results then Sydney Water would investigate and re-evaluate the option.
A sustainable solution for Sydney
At least in the short-term, and from an environmental outcome perspective, the deepwater outfalls are currently a viable option for the disposal of much of Sydney’s wastewater. However, from a water resource perspective, and because wastewater is a potential resource, Koop and Hutchings (1996) argue that “… disposal to oceans is probably not a sustainable option”. They assert that reuse and recycling of the wastewater must form part of any better use of the water resource, while conceding that community support, cost, environmental benefit, priorities, timeframes and different engineering options all need to be considered.
It should be noted that reuse was considered as part of the original disposal concept, but this option was not taken up owing to the cost and energy required, the large volumes of water and a lack of suitable, viable customers. This summation remains true in 2015, but the authors acknowledge that water is an increasingly critical resource. The uncertainty of a rapidly changing climate means Sydney Water will need to carefully plan for the sustainability of the water resource and wastewater treatment options.
About the authors
Peter Tate is an Analytics Strategist at Sydney Water Corporation. Peter has worked for 35 years in government, research and commercial organisations, assessing physical processes in estuarine, coastal and ocean environments. Much of his work involved the design and execution of environmental monitoring programs associated with the anthropogenic introduction of contaminants to water bodies. One of his specialisations is the numerical modelling of both positively and negatively buoyant jets and plumes.
Cheryl Marvell is Treatment Manager North, Sydney Water Corporation with responsibility for North Head Wastewater Treatment Plant. Her Sydney Water experience involves management of both Operational and Construction workforces in conjunction with most disciplines of engineering and science. Cheryl began her working career within the education system in the Faculty of Science at the University of Sydney before moving to the water industry in the early ’90s.
References
CCE (1976): Report on Submarine Outfall Studies. Report by Caldwell Connell Engineers for Metropolitan Water Sewerage and Drainage Board, July 1976, p 288.
Couriel ED & Wilkinson DL (1993): Sydney Deepwater Outfalls, Environmental Monitoring Program, Post-Commissioning Phase, Near Field Physical Modelling. (Volumes 1 & 2). Australian Water and Coastal Studies Report 93/01/06.
Hector D (2011): Sydney’s Water Sewerage and Drainage System. Journal and Proceedings of the Royal Society of New South Wales, Vol 144, pp 3–25.
Koop K & Hutchings P (1996): Disposal of Sewage to the Ocean a Sustainable Solution? Marine Pollution Bulletin, Vol 33, Nos 7–12, pp 121–123.
Lycke E, Magnusson S & Lund E (1965): Studies on the Nature of the Virus Inactivating Capacity of Sea Water. Archiv Fur Die Gesamte Virusforschung, Vol 17, Issue 3–4, pp 409–413. http://dx.doi. org/10.1007/BF01241195
Marine Pollution Bulletin (1996): Ocean Outfalls – Sustainable Solutions? (Special Edition. Guest Editors: Koop and Hutchings). Vol 33, Nos 7–12.
Philip NA & Pritchard TR (1996): Australia’s First Deepwater Sewage Outfalls: Design Considerations and Environmental Monitoring Performance. Marine Pollution Bulletin, Vol 33, No 7–12, pp 140–146. http://dx.doi.org/10.1016/S0025-326X(96)00165-8
Roberts PJW (2010): Underwater and Under Used – The Case for Marine Outfalls in Wastewater Disposal. Water, 21, pp 22–26.
Tate PM & Middleton JH (2000): Unification of Non-Dimensional Solutions to Asymptotic Equations for Plumes of Different Shape. Boundary-Layer Meteorology, Vol 94, Issue 2, pp 225–251. http://dx.doi. org/10.1023/A:1002431928523
Tate PM & Middleton JH (2004): Buoyant Jets of Elliptic Shape – An Approximation for Duckbill Valves. Journal of Hydraulic Engineering, Vol 130, No 5, pp 432–440. http://dx.doi.org/10.1061/(ASCE)0733-9429(2004)130:5(432)
Water Board (1990): Sydney’s Ocean Outfalls for Sewage Disposal. Planning and Implementation. Water Board Report, p 28.